There is no doubt that the philosopher Auguste Comte (1798-1857) had a great respect for science, and devoted much time and effort to meditating on it, which did not prevent him from making one of the most elegant ridicules, so to speak, in the entire history of science and culture.
Comte is today regarded as the father of positive philosophy, to which he devoted a six-volume work entitled A Course in Positive Philosophy published from 1830 to 1842. But despite all this respect for science, despite all this reflection on its development, Comte did not fully understand it, and he made one of the usual mistakes that people make about it: assuming that one is witnessing a kind of end of science, witnessing the moment when science has reached a certain degree of exhaustion from which there is little more left to discover. All of which led Comte, as we said before, to make one of the most elegant ridicule in the history of culture. In one of the volumes of his Course in Positive Philosophy, Comte asserted: “Although it is conceivable to determine the shapes, sizes and motions of the stars, we shall never succeed in studying by any means their chemical composition or their mineralogical structure”. Almost before Comte intended to bring these doors into the field of science, there were already locksmiths who were ready to burst them, using, to Comte’s mortification, a lock-pick that went by the ghostly name of “spectre”.
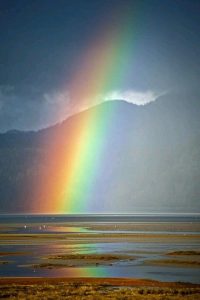
It was Newton who came up with the name “spectrum”. One of his great successes in optics was the discovery that white light was actually the jumble made up of the colours of the rainbow. When he published his first scientific paper in 1671 on the nature of light and colours, Newton called this dispersion of white light into the various colours of the rainbow a “spectrum”, a term he used again when he published his Optics in 1704. But it was almost another century before someone noticed that there was more to the spectrum of a ray of white light. That someone was William Wollaston, a compatriot of Newton’s, who noticed that among the colourful spectrum of white light, a number of mysterious black lines of varying thickness could also be distinguished; he reported the matter to the Royal Society but did not think much more of it.
The one who did was Joseph von Fraunhofer (1787-1826), a Bavarian glass industrialist. At the age of fifteen, Fraunhofer’s glass factory collapsed, with such good fortune that Maximilian, the then Prince Elector of Bavaria, took part in the rescue operations, eventually taking the injured boy as his protégé. Newton’s discoveries about the colour dispersion of white light by a lens had a threatening consequence for refracting telescopes: by using lenses to concentrate light, the refraction of white light in them produced a chromatic aberration that resulted in a loss of sharpness of the images. For this reason, Newton decided to build and recommend reflection telescopes for observing the heavens. Chromatic aberration was not inevitable, but, as it was eventually discovered, it required careful lens manufacturing to correct, something that was important not only for astronomy but also for armies that increasingly used optical equipment for mapping and surveillance. At the turn of the 18th to the 19th century, the English had a head start in this area, something that evidently worried Napoleon. Fraunhofer’s benefactor Maximilian was a good ally of the French, not least because Napoleon elevated Bavaria from an electorate to a kingdom in 1806. Maximilian proposed to help him with the manufacture of lenses, and it was precisely in this field that his protégé excelled, and despite his short life, he became an excellent glass industrialist, and his lenses were some of the best and most sought-after of the time.
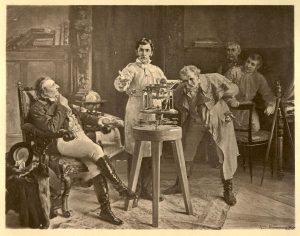
In his study of lenses, Fraunhofer soon came across the spectral lines discovered by Wollaston but, thinking that his knowledge could be used to improve crystals, he decided to investigate them. To this end, in 1814, he built the first spectrometer, a device specially designed to separate spectral colours and to show the black lines inserted between them. This instrument was as important to spectrography as Galileo’s telescope was to astronomy. Fraunhofer discovered that there were many more spectral lines in sunlight than the seven discovered by Wollaston, and also that in other types of light – that produced by sodium burning lamps, for example – in addition to the black lines, other very bright lines appeared in the spectrum.
Fraunhofer died convinced that spectral lines, both black and bright, were an intrinsic property of light, but he failed to take the definitive step that would make the spectrum a major tool of both chemistry and astronomy.
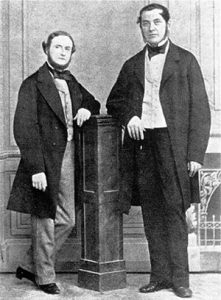
This honour was left to his compatriots the chemist Robert Bunsen (1811-1899) – who lent his surname to the name of the essential lighter for chemical practices of his invention – and the physicist Gustav Kirchhoff (1824-1887). By 1860, they had discovered that spectral lines are, in fact, a characteristic property of the chemical element that produces the light, a kind of fingerprint that makes it possible to recognise it wherever the light emitted by that element reaches – when it burns, for example. For a start, this allowed them to identify two new chemical elements, caesium and rubidium; their names derive precisely from the spectral lines that allowed Bunsen and Kirchhoff to discover them: the bright blue lines characteristic of the former suggested using the Latin word for sky blue, caesius, while the red lines of the latter suggested using rubidus, the Latin word for reddish gold. Caesium and rubidium were followed by a few more new elements discovered by various chemists in the following years using spectral techniques.
But Kirchhoff did more, first he properly interpreted the difference between bright and dark spectral lines. To understand this, one has to remember that light is an electromagnetic wave and that each colour corresponds to a certain wavelength – the distance between two consecutive peaks of the wave. The lines of the spectrum of a beam of light are precisely related to the wavelengths of the different rays that make it up, which is the same as saying the different colours that are mixed in that beam of light. Thus, as Kirchhoff explained, the bright lines of a spectrum form what is now called the emission spectrum, and relate to the colours of the light emitted by a given chemical element, while the dark lines form the absorption spectrum, which corresponds to the colours that a given element absorbs when light passes through it. Kirchhoff also established that an element absorbs precisely the same colours that it emits, i.e. its absorption spectrum is like a negative copy of its emission spectrum.
With all this, Kirchhoff dared to do something that his colleague Bunsen, a generation older, was somewhat embarrassed to do – “Lest people think we are crazy”, as he himself confessed –: he decided to study what the spectra of light from the Sun and other stars said about the chemical composition of those stars. Like most things in science, this is much easier said than done, and in any case it is a highly sensitive question involving many factors. Consider the light coming from the Sun. It involves, on the one hand, what is called the continuous spectrum produced by the solar core under great pressure and very high temperatures – the continuous spectrum is the range of colours revealed by Newton using prisms, to put it briefly but somewhat imprecisely – and the emission spectrum produced by the gaseous and incandescent solar atmosphere, although cooler than the core – the bright lines found by Fraunhofer sandwiched between the colours -. On the other hand, as this light passes through the atmosphere, the elements in the atmosphere absorb some of the colours, producing an absorption spectrum – the black lines discovered by Wollaston. Now, this absorption is a negative of the emission spectrum of the elements in the atmosphere, and this is known because we know what elements are in the Earth’s atmosphere. By carefully analysing all these ingredients in the spectrum of light from the Sun, it was eventually discovered in the early 1860s that there was hydrogen in the Sun, as well as iron, sodium, calcium, magnesium, and other elements present in the Earth – although the proportion in which these elements occur was not known until six decades later. Similar conclusions were reached by studying the spectra from the stars Aldebaran and Betelgeuse. But something else was discovered. Specifically, in a spectral analysis carried out by the French astronomer Pierre Janssen (1824-1907) on the occasion of the solar eclipse of 8 August 1868, he discovered certain spectral lines that did not coincide with any previously known element. He deduced that they corresponded to an element available in the Sun but not on Earth, which he called “helium”. Almost thirty years later, by spectral analysis of a uranium ore, it was discovered that helium was also present on Earth.

Spectroscopy was refined, especially with the introduction of photographic procedures – which allowed the study of light from a specific area of the spectrum – and other technical advances such as the spectroheliograph. This meant that by the end of the 19th century, more than 14,000 spectral lines in sunlight had been discovered, with the possibility of analysing each one specifically. All this information helped to reveal some of the innermost details of the personality of that great and classic character of the cosmic story that is the Sun.
So it took less than three decades for science to reveal Comte’s prophecy about our eternal ignorance of the chemical composition of the stars. Half a century later, a new physical theory called quantum mechanics would explain why each chemical element has a different spectrum, but that is another story that Renato is telling us.
And Hilbert? – more than one reader will ask. I will talk about Hilbert’s ridicule, which is Comte’s on the other side of the mirror, in the second part of this entry.
References
A.J. Durán, El universo sobre nosotros, Crítica, Barcelona, 2015.
Leave a Reply